From the boat, there’s nothing remarkable about the place — just choppy water and a white mooring ball, a few miles offshore. But once we’re underwater, I can see the rows and rows of PVC trees, suspended above the sand in a grid that stretches away into the distant murk.
This is a coral nursery. Each tree bears a ripening crop of a hundred or more pieces of coral. The smallest fragments are pinkie-sized, twirling on their tethers as other divers kick by; the largest hang like many-limbed chandeliers, turning slowly in the current. When I get closer, I can see the individual polyps, the anemone-like creatures that make up each fragmented colony. Nestled in the crenellated openings in their solid skeletons, they are waving their fleshy tentacles through the water, feeding.
We are there to tend the trees: Each one needs to be surveyed, scrubbed of algae, and have its biggest corals subdivided into new cuttings. Any coral bleached by high temperatures or damaged by disease needs to be removed to a nearby heap. This is a daunting task considering that the Coral Restoration Foundation (CRF) maintains five coral nurseries with some 40,000 corals growing on trees just like these ones, all along the spattered arc of the Florida Keys.
Exaggerating her gestures, a woman named Patty shows us — a group of long-time volunteers and visiting divers — how to hang new cuttings in place of the corals we cut down. We exhale long streamers of air back toward the surface, and move slowly as we pass the shared pliers around, cutting and crimping monofilament with the slow-motion care of astronauts maneuvering in zero gravity.
I pick up a piece of coral, maybe eight inches long and forked at both ends. It’s a deep amber, except for the bright white tips of its branches. It’s hard to tell how heavy an object is underwater, but this one feels light: A small thing to pin the reef’s future on. I tag it with the name and number of its tree, and drop it into a milk crate with the others we’ve harvested.
Now it’s time to go plant a reef.
I got here with a question: What happens to coral reefs as the ocean warms and acidifies over the coming century? Corals might seem removed from human society, but in fact that not-so-simple question leads to an even scarier follow-up: What happens to us?
Coral reefs are the richest ocean ecosystems we have — they support an estimated 25 percent of marine species. According to a 2014 report from the Food and Agriculture Organization, reefs are responsible for 17 percent of the protein we consume globally; in some coastal or island countries, like Sierra Leone or the Maldives, that number can be as high as 70 percent. Reefs serve as offshore larders, nurseries for important commercial fish (like tuna), and shelter for important ecological species (like sharks). They’re storm breaks for populated shores and bait for tourists whose dollars support entire local economies.
We know that reefs are crucial to the oceans as we know them, and to our societies as we’ve made them — and we know they are in serious trouble. According to one 2000 report, we’ve lost 27 percent of coral worldwide, and stand to lose another 32 percent in the next 30 years.
What we don’t know is if there’s anything we can do about it. Luckily, that won’t stop people from trying: Around the world, a scrappy handful of scientists, entrepreneurs, and volunteers are taking on this colossal problem, one branch at a time.
From regrowing the reefs we’ve already lost to engineering new corals that may be able to survive hotter, more acidic seas, these innovators are fighting everything from nutrient pollution to overfishing to ocean warming itself. If we want to succeed, we might have to learn how to rebuild an entire ecosystem from the bottom up.
Restoration
What is a coral, really? It’s an animal, but it is also plantlike, an alien amalgam of many clades. It is made up of minuscule individuals and yet its colonies comprise the largest living structures in the natural world.
Zooming in reveals the tiny components and processes that make up the organism: the fleshy coral polyp within its calcium carbonate skeleton; the microscopic algae that live in symbiosis inside the cells of the animal; the complex microbial communities that live in and around all of them. You’d also observe the way ocean chemistry interacts intimately with the creatures, changing their ability to grow, or the way a half-degree difference in temperature can upset their delicately balanced cellular machinery.
Zooming out to the reefs themselves reveals geological structures reaching up from the seafloor, growing from millions of tiny mouths and built on the compiled skeletons of previous generations. These house fish and sea urchins that graze on the algae that might otherwise overgrow the corals, larger fish that eat them, and species like sharks, whose existence marks a well-balanced ecosystem and whose absence indicates disorder. Other organisms prey on the coral itself: The crown-of-thorns starfish is so fecund and insatiable that a single individual can populate whole square miles of reef, stripping the coral of its polyps and leaving dead, white skeletons behind.
Humans have a way of messing with coral at both ends of the scale, from altering the very chemistry of the water they grow in, to destroying thousands of miles of reef by dredging, polluting, overfishing, and just generally overloading them.
But some people think that in order to fix coral, we’ll have to keep meddling. We’ll just have to do it better.

The Coral Restoration Foundation’s nursery is made up of PVC-pipe “trees” where corals grow quickly in the nutrient-rich current.Coral Restoration Foundation
Ken Nedimyer, founder of the Coral Restoration Foundation, leans hard on the coral-as-trees metaphor. If you can regrow a forest from a nursery, he thought, why not a reef? Aside from a few frustrating particulars and unknowns about coral husbandry, the model made sense to him. But no one had tried it.
In 40 years of diving in the Florida Keys, Nedimyer has watched the place change into something unrecognizable: “The reefs I saw in the early ‘70s were just fabulous. I remember going to Carysfort, and it was just acres and acres of elkhorn coral, coming right to the surface. You couldn’t swim over it; you couldn’t see the end of it. Most of the reefs in the Florida Keys were like that.”
As the stepping-stone islands filled up with people, the surrounding seas filled up with their boats and sewage. Until very recently, wastewater from the Florida Keys was flushed right out to sea or pumped into leaky septic systems. As a result, all those spectacular near-shore reefs that had made the place famous suffered a 30-year-long string of disease outbreaks, algal blooms, dredging, damage from boats, and overfishing. Today, the Carysfort Reef has mostly turned to skeletal rubble, overgrown with algae and largely empty of fish.
The problem with coral in the Keys is not unique in the Caribbean. A comprehensive study of the Caribbean’s reefs from 1972 to 2012 found that coral declined by more than 80 percent, with most of that loss concentrated in the ’80s and ’90s. An outbreak of White Band Disease knocked out almost all of the region’s staghorn and elkhorn corals — the branching corals that make up the bulk of the region’s reef structure. Another disease wiped out the local sea urchins that kept algae in check. Then in 1997 and 1998, the strongest-ever El Niño observed to date hit, and ocean temperatures shot up. Caribbean corals, struggling in the hot water, expelled their symbiotic algae and slowly starved to death, in some of the worst bleaching events in the history.
In the late ’90s, a series of papers proclaimed the end of reefs, eventual or imminent. Even if we could stop dumping so much (literal) shit and sludge on the reefs, we almost certainly couldn’t stop the global warming that had already started raising ocean temperatures above the threshold at which coral survival seemed impossible. Surely these fragile creatures, the foundation for so many critical ecosystems, would not be able to exist in that hot future world. Surely, they were all doomed.
But what seemed like a foregone conclusion to pessimistic scientists seemed like a call to arms for others. Nedimyer was of the latter. An aquarium collector, he spent most of his time underwater, catching tropical fish to sell to public and private aquariums. When an elkhorn coral started to grow on some aquarium “live rock” he was tending, Nedimyer had an idea. While elkhorn is a federally protected species in the Caribbean, the law was that anything growing on Ken’s live rock — including elkhorn — belonged to him. He could do what he wanted with it.
Nedimyer knew he could probably make a tidy fortune by growing the rare coral to sell cuttings to aquariums. But he wanted to do something bigger. So Nedimyer and his 13-year-old daughter started with a 4H project, fragmenting the original coral to grow each piece into a new colony. They were practicing for the nursery Nedimyer had pictured, an enormous underwater forest capable of restoring the Caribbean’s reefs to their former state, the way he had known them 40 years before.
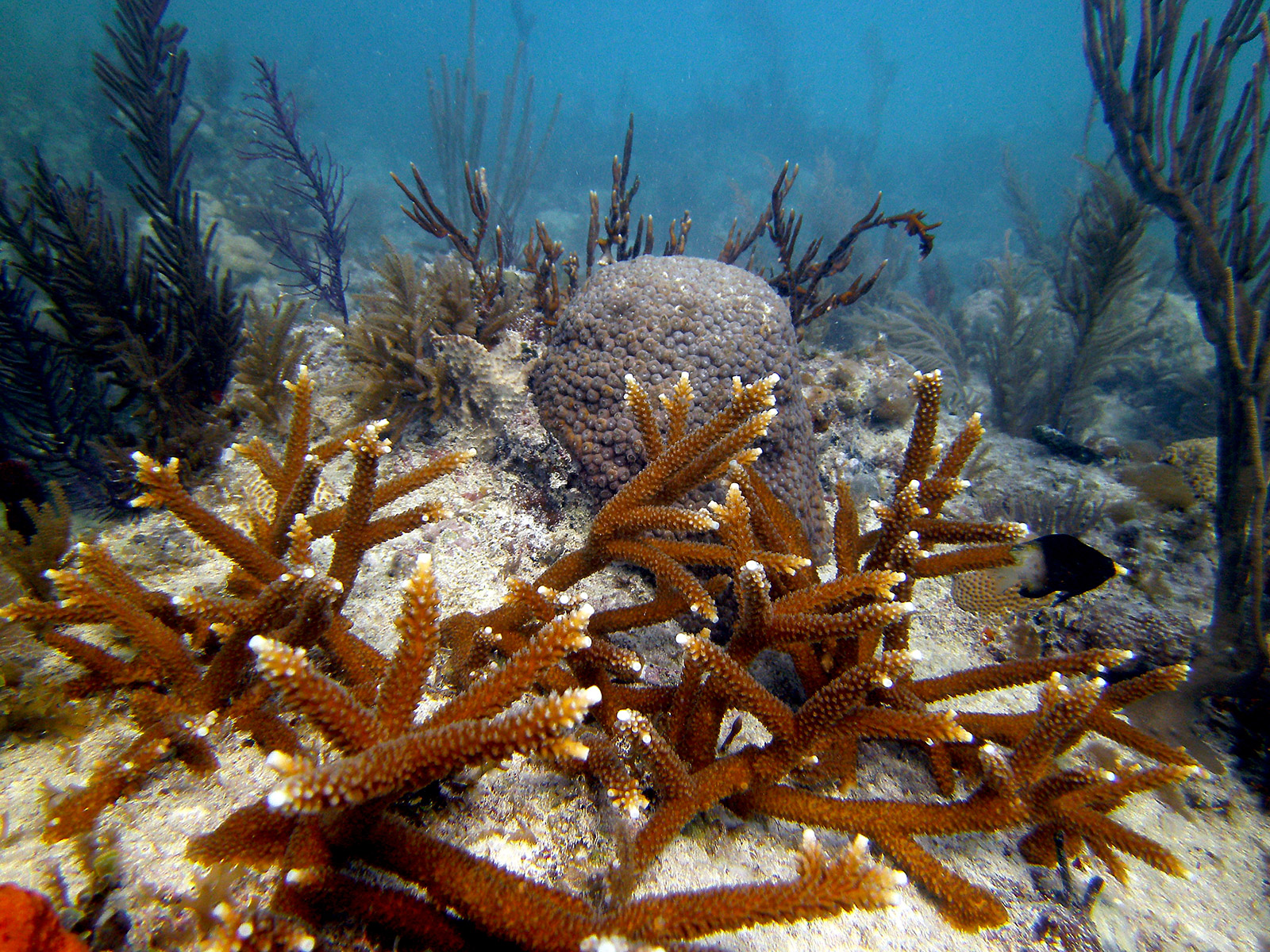
A thicket of transplanted staghorn coral takes root in the Florida Keys.CRF
In 2003, Nedimyer got federal permission to plant six corals in a spot on Molasses Reef in the Florida Keys. In 2007, after a string of bad luck and hurricanes that kept them off the reef, they planted 18. In 2015, Nedimyer and the small staff of the Coral Restoration Foundation, along with a legion of volunteers, have planted more than 18,000 of them.
Jessica Levy manages the CRF’s restoration program, which means she is out on the water most days — gathering corals from the nurseries, monitoring for signs of trouble, and planting new batches of coral on the reef. The corals she works with are the offspring of wild patches of elkhorn and staghorn that survived the mass die-offs of the last few decades.
“If they’ve gone through bleaching events and survived, if they’ve gone through cold events and survived, they’re a stronger coral,” Levy says. “They’re the ones that you want to fragment and grow and plant back on the reef. The idea being, if they survived it then, they’ll survive it now.”
In the nursery, coral grow two or three times faster than they grow on the reef. Suspended in the water column, they can gather nutrients from the current and grow in all directions without expending energy to affix themselves to the reef. Once planted, they spend a few months stabilizing, forming new tissue over the epoxy used to cement them in place. Then they start growing again.
Since they’re planted in groups of the same genotype, the corals grow together as they get bigger, forming tangled thickets that provide the kind of shelter and support a reef so badly needs. Levy says that, so far, about 80 percent of the transplants are still alive after two years on the reef. Impressive results, especially considering no one has ever tried a project like this at scale before.

A volunteer coordinator for CRF readies a boatload of divers.Grist | Amelia Urry
Nevertheless, Nedimyer and Levy and the rest of CRF know they are facing a Herculean task if they expect to replant all of Florida’s reefs by hand. But, luckily, they don’t. Even the reefs where they’ve been planting corals since the beginning are still mostly bare, featuring the odd thicket of staghorn or stubborn boulder coral.
Levy says CRF’s real goal is not to repopulate the reefs coral by coral, but to preserve the genetic diversity the species needs to stay resilient. By gathering corals from the reef, CRF can save genotypes that might otherwise be lost from the wild. Eventually, if CRF can just boost population density a little, the corals can start reproducing on their own again.
“One of the biggest obstacles is that as these populations stand now, when they do go to spawn, they’re so far apart,” Levy points out. Corals are hermaphroditic, producing bundles of both sperm and eggs during a yearly spawning event, but elkhorn and staghorn can’t fertilize themselves. They trust that their spawn will mingle in the water column, meet other coral spawn, fertilize, and avoid being eaten long enough to settle out and start growing new coral. But right now, says Levy, “these guys are so fragmented that there’s no chance of meeting up.”
“It’s a big ocean. The goal was never to outplant every coral on every reef. That’s not sustainable,” Levy says, on a rare day in her office on land. That’s the part that can be hard to explain to people, who can’t see the point of scattering a few thousand corals across reefs that once hosted millions. “The goal is to get genetic diversity out there, to the point where they’re naturally reproducing. Basically, to boost natural recovery.”
If that sounds like a big gamble to you — it is. But it might be our best bet.
To plant a coral, you first have to clear a place for it. You do this (I am a little alarmed to learn) with the chisel end of a hammer.
The day after we gathered our coral cuttings from the nursery, we go out on Molasses Reef, one of the most visited reefs in the Keys. I take a hammer from Patty and tentatively begin tapping the reef substrate, a compressed residue of coral rubble and sand, riddled with strange, iridescent sea worms.
This is not easy. Picture an astronaut trying to hammer a nail in space: For every fraction of an inch the nail goes in, the astronaut floats back a foot. In my case, each careful chisel blow to the rock sends me drifting upward like an untethered balloon. To stay down, I have to direct my head and all my attention downward while kicking steadily, as I scrape the rock clear of its algal fuzz.
I brace my coral firmly against a spot on the cleared substrate with one hand, while with the other I pinch off small globs of purple epoxy. Each branch gets a dab, and the whole thing goes down onto the spot I’ve cleared.
At least in theory: Between my own drift and wafting clouds of sediment from the divers chiseling on either side of me, it takes some maneuvering before I can get my branch of staghorn anchored firmly to the rock. To test its structural integrity, I wave my hand over it like I’m trying to fan out a flame. Sediment flies up in a cloud, but my coral — it stays put. Success.
It takes about 40 minutes for four of us to plant all 10 corals in one thicket, an area easily encompassed by a hula-hoop. As all the groups start to ascend, I can’t help but think that it doesn’t look like much. Together, we have managed to plant a single milk-crate’s worth of corals over a badminton court-sized patch of seafloor. This on the bare, rocky remains of a reef that stretches to the limits of visibility.
But something happens as we continue to rise. Fish dart in to fill the space we have made, rushing to gulp the worms we’ve dislodged in all that scraping and hammering. Suddenly, our sad jumble of coral looks a lot more like a habitat.
From 20 feet above the sea floor, I can see thickets of staghorn planted in the previous year or so. I can see how the disparate pieces of coral have grown up and together, knitting their branches into a kind of a dome. I can see, finally, where they have grown over the epoxy lumps that first sealed them to the reef. They have already taken root.
Selection
On a tiny island called Ofu, part of American Samoa just south of the equator and east of the International Date Line, corals are doing something that might have once seemed impossible.
Under the intense heat of the southern sun, in a shallow lagoon where water temperatures can reach 95 degrees F, coral reefs are thriving. While corals elsewhere struggle when water temperatures reach the mid 80s, these equatorial corals can handle the extra degrees without breaking a sweat. Those are the kinds of water temperatures people expect to see by the end of this century, which means, in a sense, Ofu’s corals are already living in the future.
Marine biologist Steve Palumbi wants to find out how these corals are so chill about extreme heat. A researcher at Stanford University’s Hopkins Marine Station, Palumbi wanted to know if these outlying “supercorals” could hold the key to a secret resiliency available to all corals.
“Corals live in a very variable environment and they have for millions of years,” Palumbi tells me one afternoon, in his office in Monterey, Calif. “So it stands to reason that perhaps, in corals that live right now, there are already some that have adapted to live in future environments.”
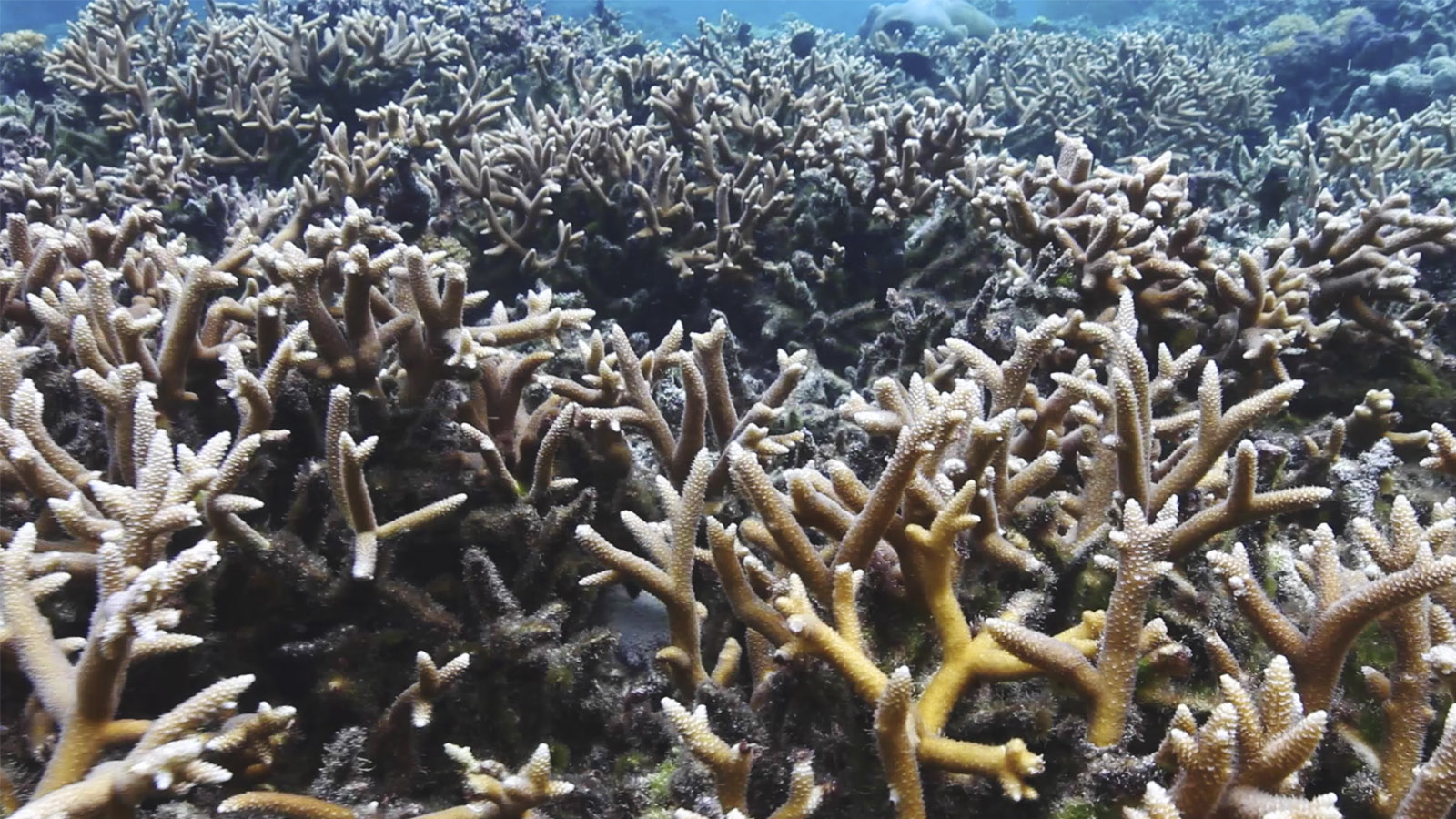
Thickets of coral growing in the super-heated water off of Ofu, in American Samoa.Dan Griffin, Garthwaite Griffin Films
So Palumbi took a team of researchers and students to Ofu to put the supercorals through their paces. He chose to focus on a type of Acropora — the same genus that includes elkhorn and staghorn corals — called tabletop corals. As their name suggests, they grow in broad, table-like ledges, gathering as much solar energy as they can and providing shelter and shade for other species.
Fast-growing and generally susceptible to heat extremes, Acropora are both a fundamental builder of reef architecture and usually one of the more fragile species on the reef. If Ofu’s tabletops seemed hardier than usual, Palumbi was determined to find out why.
Using portable water coolers (the kind you’d use to stash a couple six-packs at a picnic) rigged with heating and refrigerating elements, the researchers took fragments of coral from the reef and subjected them to a uniform battery of stress tests. As the temperatures inched up, and the corals became more and more stressed, they started to expel the photosynthetic algae they need to live, and eventually bleached.
Here’s what’s interesting: The corals the researchers chose for testing came from different parts of the same reef, some of which experienced much higher temperatures on a regular basis. These “warm-adjusted” corals were much less likely to bleach under high temperatures than those that came from cooler parts of the reef.
There are two mechanisms by which these corals (or any organism really) can adjust to an extreme environment — increased temperature, for example. The first, acclimation, is a short-term process whereby individual organisms change their physiology to become more heat tolerant. Then there’s adaptation, the longer-term process of natural selection whereby certain individuals pass on the genetic traits that make them more successful in warmer environments.
In other words: Acclimation is the tan you get on vacation, while adaptation is the skin tone you’re born with. In both cases, having more pigment in your skin reduces your risk of getting sunburned. So an organism’s life history might approximate some of the advantages of a genetic predisposition, through different mechanisms.
With corals, the combination of the two factors is more powerful than either on its own. To test this, Palumbi’s team transplanted corals from the cooler part of the reef to the warmest pool. They left them there for three years, after which the researchers subjected them to the same set of stress tests they’d undergone three years earlier, raising the temperature to an extreme level for several days at a time.
This time, the cool-adapted corals that had spent the last three years acclimating in warm pools did noticeably better than their cold-water clones. But the original warm-pool corals still outperformed their acclimated cousins, suggesting some older genetic advantage was also at work.
The next step, Palumbi says, is to start trying to use these supercorals to help corals in general. To that end, his PhD student, Megan Morikawa, is establishing a coral nursery off the coast of Ofu, where she hopes to cultivate the most resilient, heat-tolerant corals they find. Like Nedimyer, they want to replenish reefs with corals, and with the genetic diversity that will allow them to survive in a range of environments.
It’s too early to know if this will work. Palumbi is hopeful that something can be done, but aware of the challenges in scaling this kind of experiment up to a level where it can actually be effective.
“You can’t go to a replanted reef,” he says in his office, thousands of miles away from Ofu. “You can go to a replanted forest, but as of now, there is no replanted reef.”

Grist | Amelia Urry
When a coral bleaches, it doesn’t necessarily die — which means some interesting things can happen after a bleaching event sweeps a reef.
A coral polyp gathers some of its food from the water current, but it gets most of its energy by harvesting the sun’s energy, using tiny algae called zooxanthellae that live inside its cells. As the water heats up, those little algae start to produce chemicals that irritate the coral cells. As these so-called “free radicals” build up, they start to damage the cell until the coral polyp gets so agitated it expels most or all of its zooxanthellae.
From then, the coral polyp is essentially on its own. It loses the pigment it gets from its symbiont algae, turning pale white, and loses the energy it gets from photosynthesis. Without that energy, the coral feeds on its reserves and strains what food it can get from the water column. It stops growing and is at risk of eventually starving to death.
If the temperature drops again, the polyp can take up new zooxanthellae from the water and keep growing as before. But the longer the coral stays bleached, the less likely it will be able to recover.
However, there is a secondary effect to all this bleaching and unbleaching, which Palumbi and others have seized on as a possible source of hope. After a coral has bleached once, the next time it is subjected to abnormally high temperatures, it is much less likely to succumb.
In the same El Niño season that wiped out Florida’s corals, the reefs around the tiny island nation of Palau bleached extensively. Today, nearly 10 years later, they have almost completely recovered, Palumbi says. In fact, at this point most reefs in the world have bleached, he says. And a good number of them have recovered.
So corals are resilient, maybe more than we thought, and can recover from near-fatal events. But how fast can they keep up? In all their history — and at 400 million years, it’s a long one — the organisms have never experienced a rate of change like what they are subjected to today.
What’s more, a coral’s vulnerability to and recovery from bleaching have a lot to do with the presence of other stressors, Palumbi points out. With pollution, habitat destruction, overfishing, sedimentation, and so on, what would be a tolerable amount of climate change can become an intolerable amount.
In this sense, Ofu is a lucky place to be a coral. Sure, the corals living in the sheltered lagoons of the remote island may have to put up with 95 degrees F — but they don’t have to deal with many humans.
Corals can adapt and acclimate to higher temperatures — but only to a point. Even Palumbi’s supercorals, subjected to monstrously high temperatures in the stress tanks, have a limit. Eventually, given enough global warming, the world’s reefs will find their limits, too.
“I can’t do everything,” Palumbi admits. “But if I can just help keep the ocean alive long enough for us to wean ourselves off of fossil fuels, we can make it.”
This summer, Palumbi brought 80 corals back from Ofu, small fragments from the different genotypes and environments he was hoping to test in the lab. Then all but two of the corals died when he got them to the lab, and they took their heat-resisting secrets with them. Palumbi will have to go back to the reef and start over. But he’s determined to find the corals of the world that possess the superpower to resist higher ocean temperatures, and protect them.
“Saving the ocean for the next 85 years — that’s a pretty good job,” he says. “I just need to show people that it is worth it.”
Evolution
The Australian Institute of Marine Sciences stands alone at the tip of Queensland’s Cape Cleveland and looks out over the Coral Sea, toward the Great Barrier Reef.
Inside sits an array of temperature- and pH-controlled tanks, sediment pumps, and stacks of high-octane computers. With all that tech, AIMS, a federally funded research center, can feel a little like NASA, if NASA were dropped in the middle of Australia and had its laser-assisted focus retrained on the oceans. High-security access badges: Check. Brilliant, high-powered scientists: Check. Evocative acronyms: So many checks.
The blocky brick building I come to at the end of 50 km of wallaby-strewn highway is an unassuming advertisement for the pie-in-the-sky projects unfolding inside. And so, with not a little cognitive dissonance, I sign my name on a standard-issue clipboard, and head to the world’s first Sea Simulator — the SeaSim, for short.
The 10,000-square-foot facility was built with the help of a $27 million grant from the Australian government (that’s $37 million in AUD) and the ambition to do the kinds of long-running, multi-variable experiments never before possible in run-of-the-mill marine biology labs.
The sprawling building contains an upper story with a double row of sealed rooms, each controlled by a monitor panel outside. In some, heavy-duty pumps suspend dredge sediment in tanks lit a deep dance-club blue; others hold aquariums full of crown-of-thorns starfish, nightmarish, spiny creatures whose unchecked appetites have decimated whole swaths of reef.
But downstairs, I find what I came for: an enormous, open-plan room that will hold the nine tanks of Madeleine van Oppen’s time machine.
In February of this year, Madeleine van Oppen and her colleague Ruth Gates of the University of Hawaii published a paper suggesting that we start researching whether it’s possible for humans to help corals evolve to survive in a climate-changed world.
They called it “assisted evolution,” and it was a new approach to the same old problem: Corals already possess some innate resilience, but they cannot evolve fast enough to keep up with the accelerating pace of climate change. So let’s just make them evolve faster.
With a grant from the Paul-Allen-funded Ocean Challenge prize, covering a five-year-long research project split between AIMS and the University of Hawaii, van Oppen and company set out to see if that’s even possible. If they could breed new generations of corals that outperformed their parents, maybe they could identify the secret to coral resilience, be it genetic, microbial, or something else altogether.
And for that, they need corals. A lot of them.

AIMS staff unload samples of coral and sand from the Great Barrier Reef, to be used in SeaSim experiments.Grist | Amelia Urry
The boat comes in in the afternoon. Van Oppen drives me down to the port, where researchers with a heavy crane are lifting pallets of stacked plastic bins from the deck of the dive boat into the back of a truck. It’s sunny, so they work fast — these corals have come from Davies Reef, a nearby patch of the Great Barrier, and they are likely feeling the heat.
As he sloshes excess seawater out of the bins, research manager Neal Cantin explains to me what they’ve brought back: entire tubs of sand and sediment, heaps of worn-down and overgrown old coral skeletons (or “live rock”), some algae and sponges for a different experiment, and, of course, about 80 coral colonies for van Oppen’s experiment.
The corals are the main event, but the sand and sundry are important, too. With all these ingredients — and the ambient microbial communities they host — the researchers can create a rough copy of the reef the corals just left. Then, using data from a weather tower right over Davies Reef, they can adjust the water temperature throughout the day to match natural conditions as closely as possible.
I hitch a ride back to the SeaSim with a couple of tubs of coral and live rock. There, in the largest chamber of the Sea Simulator, Cantin and a graduate student are arranging the corals in three long tanks, flushed with seawater and lit with full-spectrum LEDs that mimic sunshine. These tanks will serve as an indoor ocean to these corals for the next five years, or longer.
Eventually, there will be nine tanks in the enormous, hangar-like room, grouped by threes: One trio will be treated with water heated and cooled to match the real-time temperature data from Davies Reef. The pH levels will match normal variations the reef might experience over the course of an average day, becoming more acidic at night, in the absence of photosynthesis. The second set will have its water heated and soured to match the ocean conditions we are predicted to experience by midcentury. The third will experience the most extreme conditions, with temperatures and acidity mimicking what we might see by 2100 if climate change is left unchecked.
All the corals will spend a few months acclimating to these new normals. The scientists expect many of them will become stressed, even bleach — but that’s only the beginning. What they really want to find out is how the kids do.
The idea that humans might “assist evolution,” while it sounds wacky, is not truly anything that new. We’ve been changing the evolution of plenty of species, intentionally and otherwise, for about as long as we’ve been living in social groups. We “assist” evolution when we breed animals to perpetuate traits — milk production, for example, or docile behavior — that we find beneficial. We also can be said to assist evolution when we build environments, like cities, where certain animals, like pigeons, can find an ecological niche that never existed in the wild.
And we’re already applying selective pressures to coral. From global warming to ocean acidification, we’re changing the natural environment such that some corals are better adapted to survive than others. But evolution, while it’s happening, is not happening fast enough — and those selective pressures of ocean acidification and ocean warming run the risk of selecting reefs right out of existence.
Genetic selection is the most familiar kind of evolution, but the process of accumulating genetic adaptations is typically a slow one, reliant on chance mutations that occur in the right place at the right time. And corals only spawn once a year and grow slowly — which means there aren’t many chances to get things right.
So van Oppen’s group is looking to find the helpful mutations first, and maybe eventually find a way to spread them among wild corals. She is also looking for “epigenetic” changes in the corals, which is a catch-all term for the things that act on the genome, but are not a part of it.
For example, certain molecules may be attached to parts of an organism’s DNA, controlling whether a certain gene is turned on or off. If the gene is turned off, the cell won’t produce the protein that the gene codes for. In this way, an individual of a species may possess all the genes necessary to produce some trait without actually expressing the trait — all depending on its epigenetics.
These epigenetic markers have, in some cases, seemed to be heritable from a parent organism to its offspring. Van Oppen believes that epigenetics may hold the key to jump-starting corals’ non-traditional evolution in the face of warmer, more acidic waters. And if we can get a head-start on climate change by seeing what these changes might look like, maybe we can help nudge today’s reefs in the direction they need to go.
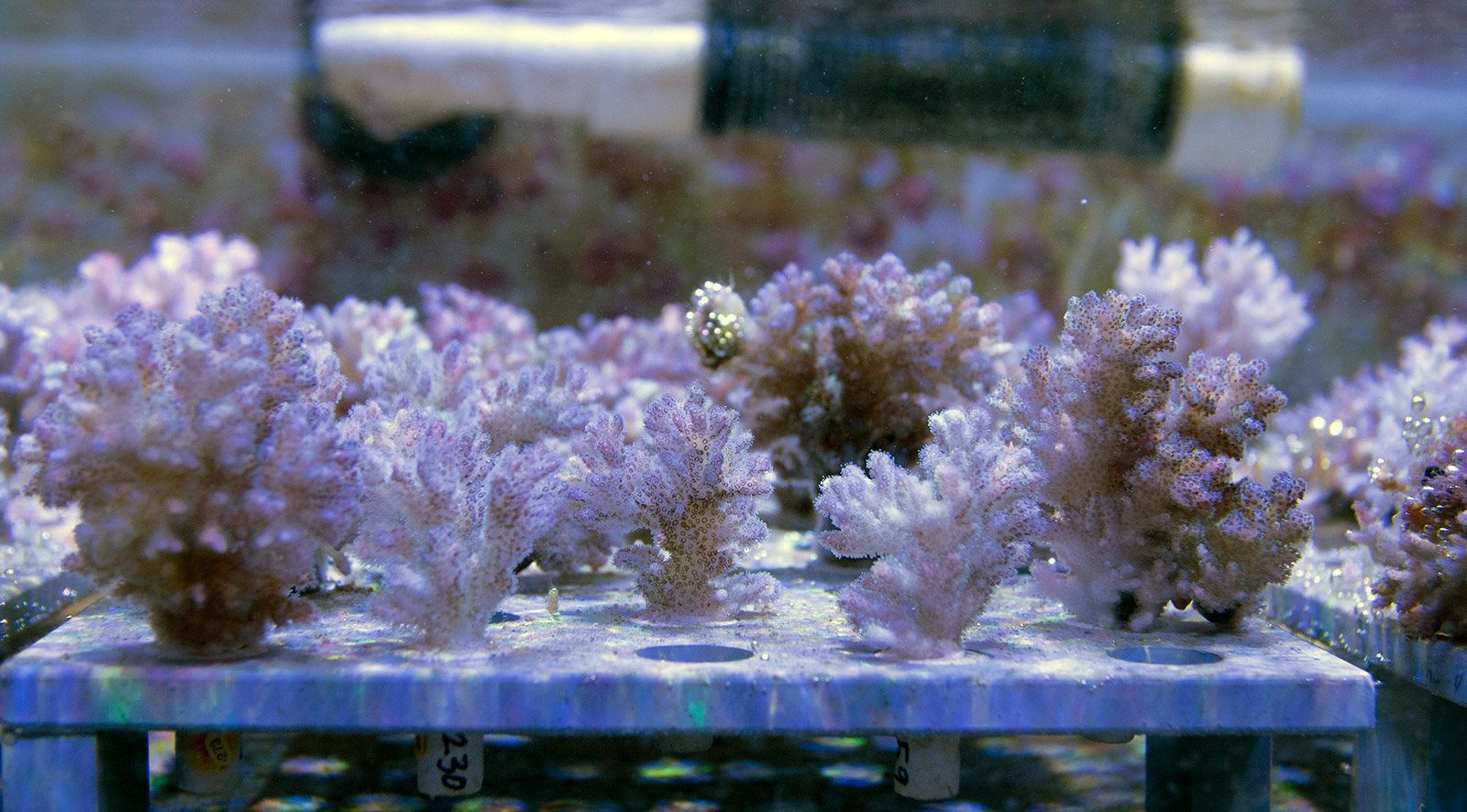
Baby Pocillopora growing up in the SeaSim tanks.Grist | Amelia Urry
Pocillopora — “poss-ill-uh-pour-uh” — acuta are pale, knobby lumps of coral, each about the size and shape of a head of cauliflower. They are fast-growing and branching, which makes them important reef building species, not unlike the more common Acropora.
But Pocillopora do one weird thing differently from most other corals. Instead of spawning just once a year, Pocillopora can reproduce asexually, releasing fully formed clonal larvae every month or so. In van Oppen’s lab, these larvae will settle onto little ceramic discs prepared by researchers and start to grow. Some of the baby corals will be moved around — the hot future larvae back to cold present-day tanks, and vice versa — to see how they do. Then this whole step will be repeated one more time, with a third generation of corals.
If the second- and third-generations of corals born and raised in future ocean conditions seem robust, that may tell us about how reefs could change in real-time. But even if corals are capable of keeping up with stressfully high temperatures and acidity, they still may change in ways that will fundamentally change the reefs themselves. The corals may not grow as fast, and they may reproduce differently. Larvae might have more trouble developing, or might develop differently.
And if the third generation of Pocillopora do end up stronger than their forebearers? The next step could be one of sci-fi proportions: to take these speed-evolved corals out onto the reef. These ultra resilient corals could potentially be used to restore damaged reefs, while providing a set of genes that could pass some of that accelerated resilience on to the next generation.
The other experiments van Oppen’s team will be running have some similarly stunning implications. For example, if the scientists experimentally evolve a strain of symbiotic zooxanthellae that makes its coral host more resistant to bleaching, they could conceivably grow huge tanks of it, to dump on reefs during especially hot spells.
To be clear, these are all pretty long shots for a lot of different reasons. But even if they’re shown to work, is it a good idea?
“It’s not up to us in the end whether it will actually be implemented,” Madeleine van Oppen told me, before I could even ask. Her experiments are such an early step on the road to large-scale reef engineering that she only spends a few minutes talking about the possibility. For van Oppen, the important thing is that we at least start asking the right questions. Answering them is a whole different research project.
What we definitely don’t want to do, she says, is to introduce new problems into a system we’ve already thrown out of whack. “We are very good at messing things up, changing this planet tremendously,” van Oppen says. “We’re also very innovative and intelligent, I suppose. We’ve come up with lots of good solutions.”
One thing is sure: We’ll have to put the brakes on climate change eventually, if we want to stave off disaster — on this point, everyone agrees. But even if we phase out greenhouse gas emissions in the next 10 or 20 years, the atmosphere and oceans will continue to warm for years after — so we need to be prepared to deal with that.
In a certain light, this crisis is nothing new for humans, says van Oppen: “We pollute the environment and then we clean it up. We develop bacteria that can digest hydrocarbons when there’s been an oil spill. It’s no different from other things where we clean up after the mess we have made. We do that all the time. Unfortunately, that’s how humans are.”
I’m starting to get the sense that if scientists, governments, and nonprofits can figure out how to engineer a better coral reef, it will be because, in the end, it’s easier to change nature itself than it is to change human nature.
The Great Barrier Reef is a bucket list item if there ever was one. It’s the largest living barrier reef in the world, roughly the size of Italy, and one of the only biological objects visible from space.
Home to some of the strangest (and most venomous) creatures in the ocean, the GBR is a poster child of what we stand to lose as the oceans warm. I had always wanted to see it for myself.
So one morning, on an ocean as flat and blue as a swimming pool, empty of other boats all the way to the cloud-strewn horizon, I hold a mask and regulator to my face and step into the water.
Underneath, huge mounds of coral rise 30 feet from the seafloor almost to the surface. I am surprised to find that these seamounts — called “bommies” in Aussie — are veritable catalogue advertisements of reef health and diversity. I see all kinds of Acropora — big, branching thickets of elkhorn, wide terraces of tabletop coral — all colored a healthy deep orange or yellow. Pocillopora sit on the tops of lower mounts like frilly heads of lettuce. I see enormous brain corals and tiny, fine-fingered soft corals that swayed in the current.
All around these pillars and buttresses, life swarms and flashes like static electricity: angelfish, damselfish, clownfish, a massive school of yellow-finned jacks winding between two bommies. There are skates bearing blue spots the size of silver dollars and parrotfish in all the colors of an ‘80s aerobics instructor’s leotard. Electric-blue seastars stand out like asterisks on the sand, where giant clams flick their purple lips as I swim over.
If there is trouble on the Great Barrier Reef — and, by all accounts, there is — I can’t see a sign of it from behind my mask. In all its wild wholeness, this little patch of Edenic reef seems to stand both for what has been lost everywhere else, and what we stand to save.
But as I haul myself back out of the water — suddenly transformed back into a clumsy two-legged creature wearing 60 pounds of dive equipment and a fogged mask in front of my face — I feel both smaller and bigger than before. No matter how much I wanted to believe it while I was underwater, I knew that the idea of a pristine reef is an illusion. There are no virgin reefs to “preserve.” Any reef, any ecosystem, no matter how lovely, is in the process of being affected by the 7 billion (and counting) people of the world.
But that’s the way it’s always been. The reef is always changing: It is fed by currents and smashed by storms, and subject to the whims of the animals moving in waves over it. Flux is a fact of nature, and humans are now a part of that. If we want to start setting right some of the balances we’ve upset, coral reefs are as good a symbol as any of where our real work begins — at the intersection of the world and ourselves.
This story was supported by a grant from the Earth Journalism Network.
